Issue |
Dairy Sci. Technol.
Volume 89, Number 3-4, May-August 2009
1st IDF/INRA International Symposium on Minerals and Dairy Products
|
|
---|---|---|
Page(s) | 405 - 416 | |
DOI | https://doi.org/10.1051/dst/2009016 | |
Published online | 20 May 2009 |
Note
Changes in milk composition and processing properties during the spring flush period春季牧草期乳组成和加工特性的变化
Changements dans la composition et les propriétés technologiques du lait lors de la période de mise à l’herbe
Helen Grimley, Alistair Grandison* and Michael Lewis
Department of Food Biosciences, University of Reading, Whiteknights, P.O. Box 226, Reading RG6 6AP, UK
* Corresponding author (通讯作者): a.s.grandison@reading.ac.uk
Received: 29 September 2008
Accepted: 28 March 2009
The period, known to UK farmers and processors as the “spring flush”, when the cows’ diet changes from dry feed to spring pasture, has long been established as a time of change in milk properties and processing characteristics. Although it is believed to be a time when problems in processing are most likely to occur (e.g. milk that does not form clots or forms weak gels during cheesemaking), there is little evidence in the literature of detailed changes in milk composition and their impact on product manufacture. In this study, a range of physicochemical properties were analysed in milk collected from five commercial dairy herds before, during and after the spring flush period of 2006. In particular, total and ionic calcium contents of milk were studied in relation to other parameters including rennet clotting, acid gel properties, heat coagulation, alcohol stability, micelle size and zeta potential. Total divalent cations were significantly reduced from 35.4 to 33.4 mmol·L−1 during the study, while ionic calcium was reduced from 1.48 to 1.40 mmol·L−1 over the same period. Many parameters varied significantly between the sample dates. However, there was no evidence to suggest that any of the milk samples would have been unsuitable for processing – e.g. there were no samples that did not form clots with chymosin within a reasonable time or formed especially weak rennet or acid gels. A number of statistically significant correlations were found within the data, including ionic calcium concentration and pH; rennet clotting time (RCT) and micelle diameter; and RCT and ethanol stability. Overall, while there were clear variations in milk composition and properties over this period, there was no evidence to support the view that serious processing problems are likely during the change from dry feed to spring pasture.
摘要
奶牛的膳食从干饲料转变为牧草饲料的时期被英国牧民及加工者称为“春季牧草期”,这个时期乳的成分及特性均会发生一系列的变化,一度认为这会在加工干酪过程中会发生不凝乳或者凝块较弱等问题,但是关于乳成分的变化及其对加工影响方面的研究却未见报道。2006年在 5 个牧场分别在春季牧草前期、中期及后期取样,分析了各个时期奶样中总钙及离子钙含量与凝乳酶凝乳、酸凝乳、热凝乳、酒精稳定性、胶束大小及 zeta 电势等各项指标之间的关系。结果表明,这一时期总二价阳离子的含量从35.4 mmol·L−1降低到33.4 mmol·L−1,而钙离子的含量从 1.48 mmol·L−1 降低到1.40 mmol·L−1,样本之间成分差异显著。但是分析结果没有表明这种乳成分不适于乳品加工,也就是在规定的时间内不发生酶凝乳或者凝块较弱以及不发生酸凝的现象。根据大量的统计数据表明钙离子浓度与pH值,酶凝时间与胶束直径,以及凝乳时间与酒精稳定性之间都显著相关 总之,尽管从干饲料到牧草饲料这个时期乳成分与特性均发生了显著的变化,但是并不会导致严重加工问题的发生。
Résumé
La période de mise à l’herbe que les fermiers et transformateurs britanniques appellent « spring flush » , où les vaches passent d’une alimentation sèche à la pâture de printemps, est connue depuis longtemps comme une période de changement des propriétés et des caractéristiques technologiques du lait. Bien que l’on sache que c’est une période où des problèmes de transformation peuvent apparaître (par exemple, absence de coagulation du lait ou formation de gels mous au cours de la fabrication fromagère), peu d’informations existent dans la littérature sur les changements dans la composition du lait et leur impact sur sa transformation. Dans cette étude, toute une gamme de propriétés physicochimiques a été analysée sur du lait collecté dans 5 troupeaux laitiers, avant, pendant et après la période de mise à l’herbe 2006. En particulier, les teneurs en calcium total et ionique du lait ont été étudiées, en relation avec d’autres paramètres incluant la coagulation par la présure, les propriétés de gélification acide, la coagulation thermique, la stabilité à l’alcool, la taille des micelles et le potentiel zéta. Les cations divalents totaux étaient significativement réduits de 35,4 mmol.L−1 à 33,4 mmol.L−1 au cours de l’étude, alors que le calcium ionique était réduit de 1,48 mmol.L−1 à 1,40 mmol.L−1 sur la même période. Plusieurs paramètres variaient significativement entre dates d’échantillonnage. Cependant, il n’a pas pu être démontré qu’un des échantillons était impropre à la transformation – par exemple aucun des échantillons ne coagulait pas à la chymosine en un temps raisonnable, ou formait des gels présure ou acide spécialement mous. Un nombre de corrélations statistiquement significatives était trouvées parmi les données incluant la concentration en calcium ionique et le pH, le temps de coagulation par la présure et le diamètre des micelles, le temps de coagulation par la présure et la stabilité à l’alcool. Globalement, alors qu’il y avait des variations claires dans la composition et les propriétés du lait pendant cette période, aucune preuve ne permettait de soutenir l’idée que de sérieux problèmes de transformation puissent survenir lors du passage d’une alimentation sèche à la pâture de printemps.
Key words: milk / composition / processing / coagulation / season
关键字 : 乳 / 成分 / 加工 / 凝乳 / 季节
Mots clés : lait / composition / transformation / coagulation / saison
© INRA, EDP Sciences, 2009
1. INTRODUCTION
There are two distinct ways of managing dairy cows, which determine the feeding regimes, and consequently the characteristics of milk and dairy products. Pastoral farming is practised almost exclusively in New Zealand, in most of Australia, and for a large part of the year in Ireland, and the cows spend their time outdoors grazing pasture. In other cooler climates, such as North America and much of Europe, it is a common practice for dairy cows to be housed indoors for most of the year and fed on concentrates and rations largely based on grains [3]. In the UK, cows are generally offered dry feed and are kept indoors during the colder winter months and are only allowed to feed on the pasture during the warmer spring and summer months. These differences in feeding affect milk yield, and the composition and other qualities of the milk.
The transition period in which the cows move from inside, and a diet of dry feed, to grazing outside on the pasture is associated with a well-established increase in milk yield [10] which is traditionally termed the “spring flush”. The transition takes place in spring but the actual timing depends on the weather. It can be a gradual process where the low-yielding cows go out during the day to begin with until eventually the whole or majority of the herd is out during the day and night. The turning out period can be as short as a week to more than a month, depending on farming methods and weather conditions.
The transition period between spring and summer has long been considered to be a period of change in milk properties and a time when a number of problems are experienced while processing milk. For example, it is well known in the industry that whipping cream properties are very variable during this period (Blackmore Vale Dairy, Dorset, UK – personal communication). Similarly, the cheese industry reports problems with extended rennet clotting times (RCTs) that can result in the disruption of production schedules, or in some circumstances, can halt production when milk fails to form a coagulum. The average protein content of milk increases over the spring period; e.g. in 2004, the average protein content of UK milk was 3.18% in March and 3.25% in May [4]. Therefore, one might expect cheese yield and processing behaviour to improve simultaneously. However, sudden changes in content of protein and other constituents, especially from smaller localised groups of animals, may lead to more sporadic changes in behaviour. Grandison et al. [7] studied the changes in the chemical composition and the renneting behaviour of cows’ milk during the transition period, in particular noting that curd firmness increased after the change to spring grazing, which was related to casein and mineral composition. However, no pattern in RCT could be established. Green and Grandison [8] discussed environmental, seasonal, lactational and dietary effects on milk composition and cheese production. They suggested that dietary factors can have an effect on the renneting behaviour of milk. Curd firmness is affected by pasture feeding and could be attributed to changes in the casein concentrations of milk. It is also suggested that changes in the climate affect renneting properties, and in turn cheese quality. The impact of the climatic changes may be due to a combination of factors, such as compositional changes in the pasture and potential stress caused to the animals during hot weather. The composition of milk, in particular fat and protein, changes during pasture feeding and this contributes to cheese yield. However, much of the data are conflicting, and it is difficult to determine the extent to which these factors actually influence cheesemaking properties. Nevertheless, the most consistent factors appear to be the high casein concentration, the low pH and the high micellar calcium phosphate associated with pasture feeding. In order to gain a clear understanding of the relation between milk composition and cheesemaking potential, a precise knowledge of the components present is required [8].
This study aims to identify the major changes taking place during the spring flush and to relate these changes to potential differences during the processing of milk obtained from commercial herds. Of particular interest in this study is the investigation into calcium content and more specifically the ionic calcium content of milk. The ionic calcium content is related to the stability of milk and can have an influence on processing and, therefore, potentially has a function in relation to the changes taking place in milk during the spring flush, and problems during the processing of such milk. In addition, this study incorporates measurement of micelle size and zeta potential in an attempt to relate chemical properties to rennet gel-forming behaviour.
2. MATERIALS AND METHODS
2.1. Milk samples
This study was conducted during the spring/summer of 2006. Samples were collected from five commercial farms every 2–3 weeks, during the period of 20th March to 12th June 2006. Two-litre samples of morning milk were collected from the well-mixed bulk tank of farms in the morning and transported to the laboratory in insulated containers within 3 h.
The cows were healthy Friesian/Holstein breed in all cases. The herd numbers ranged from 150 to 400 cows in the five farms, and somatic cell counts ranged from 150 to 300 × 103 cells·mL−1 for all samples throughout the study. No evidence of mastitis was reported in any of the herds at any stage of the study.
2.2. Chemical analysis
The pH of the samples was recorded using a portable pH meter with temperature probe for correction (Oakton Instruments, Vernon Hills, IL, USA).
Fat, total protein and lactose contents were measured using a DairyLab II analyser (Multispec Ltd., York, UK).
Urea concentration in milk was measured, courtesy of CEDAR (Centre of Dairy Research, Arborfield, Berkshire, UK), using a DairyLab II analyser with urea channel (Multispec Ltd., York, UK).
Total divalent cations (TDC) in milk samples were measured by titration against ethylenediaminetetra-acetic acid (EDTA) disodium salt, by the method of Davies and White [5]. The EDTA sequesters all divalent ions within milk and therefore will account for both the calcium and magnesium present. Since the concentration of magnesium is ~ 1/10 of that of calcium, it can be assumed that this test is an estimation of the calcium content of milk.
Ionic calcium was measured using a Ciba Corning 634 ISE Ca2+/pH analyser (Bayer plc, Diagnostics Division, Newbury, Berkshire, UK). This instrument employs potentiometric methods that measure the activity of the free calcium ions that interact with the calcium ion electrode. The Ciba analyser was initially designed for measuring the ionic calcium activity in blood samples and was modified to measure the same in milk [9]. The results are presented as mV readings. The machine was calibrated using calcium standards in the range 0.5, 1.0, 1.5, 2.0, 2.5 and 3.0 mmol·L−1. Milk samples were analysed in duplicate.
Sodium and potassium were measured in triplicate using the Rapidlab 348 pH/Blood Gas analyser (Bayer plc, Newbury, UK). The machine was calibrated using sodium chloride (concentration = 10, 20, 50 and 100 mmol·L−1) and potassium chloride (concentration = 4, 20, 40, 60 mmol·L−1) standards, and the samples were analysed in triplicate. Results in mV were converted to mmol·L−1 using calibration curves.
To measure titratable acidity (TA), 1 mL of phenolphthalein solution (0.5% w/w) was added to 10 mL of milk. This mixture was titrated against 0.11 mol·L−1 NaOH (VWR International Ltd., Poole, UK) to an end point marked by a pink colour persistent for at least 5 s. Results were expressed as percentage of the lactic acid.
2.3. Physical properties
The stability of milk to ethanol was determined by mixing equal volumes of milk and a range of ethanol solutions as described by Tsioulpas et al. [12]. Ethanol stability was defined as the highest concentration of ethanol tolerated by the milk before clots were observed.
The casein micelle diameter and zeta potential were measured using a Zetamaster ZEM (Malvern Instruments Ltd., Malvern, Worcestershire, UK). For casein micelle size, 100 μL milk was diluted with 3 mL distilled water. For zeta potential, 60 μL milk was diluted with 3 mL water.
Sedimentation was assessed in sterilised milk samples (heated to 120 °C for 15 min in 150 mL baby cans in a batch retort) by centrifuging the milk samples at 4200 rpm for 1 h. The supernatant was discarded and the pellet was dried in the oven at 70 °C for 36 h. The dried pellet was weighed and the sediment was calculated as a percentage of the milk.
The heat stability of milk was measured using an oil bath at 140 °C. The samples were checked for signs of coagulation every 2 min and the time of coagulation was recorded.
The RCT was estimated using two methods:
Manual measurement [11]: Freshly prepared 1:10 diluted chymosin (Chymax Plus, Chr. Hansen, Hungerford, UK) was combined with milk at 0.03% (v/v) and incubated at 30 °C. The time from the addition of diluted chymosin to the first sign of sudden breakdown of the film on the tube wall was measured and defined as the RCT.
Instrumental measurement: A C-VOR Torque Balance controlled-stress rheometer (Bohlin Instruments, Malvern Instruments Ltd., Malvern, Worcestershire, UK) was used with the cup and bob geometry as described by Tsioulpas et al. [12]. The dilution factor and temperature were the same as for the manual measurement. Gels were oscillated at a frequency of 0.5 Hz and with an applied strain of 5%. A circulating water bath maintained the sample at 30 °C throughout the analysis. Dynamic viscosity, tan delta, complex modulus (G*), elastic modulus (G′) and viscous modulus (G″) were measured at 30 s intervals. When clotting occurs, the viscosity of the sample increases and this is indicated by a sudden increase in the complex modulus curve. RCT is described as the point at which the G′ crosses over G″ [9].
Gelation properties of acid gels produced with Glucono-delta-lactone (GDL) were measured as follows: GDL powder (ADM Ingredients Ltd., Widnes, UK) was added to pre-warmed milk (40 °C) at 1.5% (w/v). The samples were incubated for 3 h at 40 °C and the resulting gels were cooled in ice water for 20 min. Stirred gels were produced by mixing the set gels with a SS 10 stirrer (Stuart Scientific, Stone, UK) for 150 s at 100 rpm. The gels were stored at 4 °C for an additional 2 h and 40 min. A 50-mm-diameter cylindrical probe was used to measure the compression force (N) of the set gels using a TA.XT2i analyser (Stable Microsystems, Godalming, UK), which penetrated the sample to a depth of 20.0 mm (speed = 1.0 mm·s−1).
Dynamic rheological tests were performed on the stirred gels using the C-VOR Rheometer in a controlled strain mode with the parallel plate geometry (40 mm) and 500 μm set gap. The temperature of the samples was maintained at 11 °C using a circulating water bath. All the gels were analysed by a frequency sweep (range 100 Hz down to 0.1 Hz). The storage modulus (G′) and the loss modulus (G″) were recorded, and the complex modulus (G*) was calculated as follows:
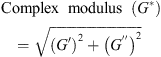
Stirred gel viscosity was hence expressed as G* (Pa).
2.4. Statistical analysis
Repeated measures analysis was carried out using a mixed model (Genstat, 11th edn., VSN International Ltd.), to investigate the significance of date and stage of spring flush in a fixed model. By subsequently dropping terms from the model, it was possible to determine which terms were required to explain the variation.
Pearson Product Moment Correlations (r) were calculated to determine any correlations within the data (SPSS 14 for Windows, SPSS Ltd., London, UK). The values reflect the degree of linear relationship between two variables.
3. RESULTS
Milk samples were collected on the same calendar dates for the five farms, and hence date is calculated as days from the first collection and is the same for all farms. However, the actual dates of turning out the animals varied between farms, and the duration of the turning out periods were different. It was possible to group the data into the three stages of spring flush retrospectively, i.e. before, during and after the turning out. The mean physicochemical data from milk samples from the five farms are presented in Table I. The TDC of the milk was high before the cows were turned out, and it gradually decreased throughout spring flush. The ionic calcium followed the same trend as total calcium. Higher calcium concentrations before turning out would be expected to coincide with higher protein content at this point, because calcium is predominantly associated with casein. However, the protein was lower before turning out, suggesting that more calcium was in the soluble phase in the milk collected before turning out. The ethanol stability of the milk decreased after turning out. Urea concentrations were highest during the time when the cows were being turned out. The zeta potential of the milk increased, becoming less negative, throughout the spring flush study. The amount of sediment formed during sterilisation decreased markedly throughout the study, to almost half the quantity after turning out, compared to before turning out. The RCT of the milk increased during the period that the cows were being turned out and then decreased after turning out, to a value similar to that of before turning out. The same trend was observed with both methods of analysing RCT. However, the absolute values for RCT are slightly different depending on the method used. This is because the two methods measure slightly different properties. In the manual method, the sample is regularly agitated and mixed and the end point is the first appearance of flocs; whereas the mixing in the rheometer is restricted to 20 s of premixing before analysis begins, and the end point is a change in viscosity. This may explain why the samples measured manually generally coagulated faster than the samples analysed using the rheometer. The data from the rheometer may be more applicable to clotting times related to cheese production as the milk is not stirred during rennet clotting in commercial practice. The strength of the set acid gels remained similar throughout the study, but the viscosity of the stirred acid gels (indicated by G*) gradually increased throughout the spring flush study.
Mean data from physicochemical properties of milk throughout spring flush.
Repeated measures analysis (Tab. II) indicated that date had a significant effect on a number of parameters. In particular, the TDC and ionic calcium levels were strongly related to date. However, stage of spring flush was only significant in the case of RCT (measured instrumentally) and lactose. Overall, the stage of spring flush was not a significant factor, therefore, suggesting that the variation in the data cannot be attributed to the spring flush per se.
Repeated measures analysis to test the effect of date and stage on variation of measured parameters.
The influence of days from turn out on raw data, for TDC, ionic calcium and some of the coagulation properties, was examined. The date on which the farmers started to turn out their cows was determined for each farm and defined as day 0. Any samples collected before this date were given negative numbers of days, and the number of days from turn out was determined for all the samples collected, retrospectively. Initially, the TDC content of the milk was high before the cows were turned out, then the levels decreased gradually from day 0 while the cows were feeding from the pasture (Fig. 1). Ionic calcium displayed a similar trend (Fig. 1).
![]() |
Figure 1. The TDC and ionic calcium content of milk from five farms throughout spring flush. |
The RCT was very variable from week to week, both within and between herds (Fig. 2). For example, the RCT of the milk from Farm E varied from ~ 600 to 1600 s during the study. The general trend for RCT was to increase following turnout, followed by a reduction in the later stages of the study. The stirred acid gel viscosity (G*) was low before turning out, and then gradually increased from day 0. Set acid gel strength (data not shown) followed a similar, though less marked, trend to the viscosity throughout spring flush.
![]() |
Figure 2. The RCT (measured instrumentally) of milk from five farms throughout spring flush (mean of two measurements) and stirred gel viscosity (complex modulus) of gels made from milk from five farms throughout spring flush. |
Table III shows that there were a number of significant correlations within the data, even though the correlation coefficients were sometimes quite low. Factors which do not significantly correlate are not shown in the table. It is not always clear which correlations are causal and which are incidental. Fat correlated significantly with a number of physical and coagulation properties, although it seems unlikely that these are causal relationships. Protein correlated significantly with the strength and viscosity of the acid gels which would be expected, although the correlation coefficients are quite low. There was a significant relationship between pH and ionic calcium, and the amount of sediment correlated with the TDC, but surprisingly, not with ionic calcium. Ethanol stability was related to a number of the parameters tested, including RCT (which seems logical as milk samples that are susceptible to coagulation by ethanol would also be expected to be susceptible to coagulation by rennet) and stirred gel viscosity. The casein micelle diameter correlated significantly with both the acid gel properties and the RCT. This is understandable as the caseins form the structure of the gels and their size could affect the strength and structure of the gels produced. The zeta potential is important in the formation of acid gels because the zeta potential of the casein approaches zero before the micelles can coalesce. Zeta potential correlates with the acid gel properties. The correlations between set gel strength and stirred gel viscosity, and between the two methods of measuring RCT are not surprising.
Pearson’s correlation matrix for spring flush data.
Acid gel strength increased significantly (Tab. III) as the protein concentration increased. Since protein is involved in forming the basis of the structure in an acid gel, the relationship is as expected. There was also a significant correlation between RCT and micelle diameter. For the casein micelles to coagulate, during rennet clotting, the chymosin must act on the κ-casein, on the surface of the casein micelles. The process of coagulation of renneted milk is a two-stage process, which involves the initial attack of the enzyme on the κ-casein and the subsequent clotting of the micelles which have been destabilised by this enzymatic attack. According to the correlation, milk containing smaller casein micelles clotted quickly suggesting that the micelles were easily destabilised. The increased clotting time of the larger micelles may be due to the comparative ease in the removal of the κ-casein, which may be quicker for smaller micelles. In addition, smaller micelles may potentially aggregate more quickly. This is in agreement with the findings of Ford and Grandison [6] who investigated the coagulation properties of large and small casein micelles produced by centrifugation of skimmed milk. Although the RCT was similar for the large and small micelles, the large micelles clotted slightly more slowly than the smaller ones. They suggested that the micelle size range in a milk sample may affect both phases of coagulation, and that it can be a determinant of clotting time and coagulum strength.
The relationship between ionic calcium and pH is shown in Figure 3. The following equation determines this relationship [13]:

![]() |
Figure 3. Relationship between ionic calcium and pH during spring flush. |
4. DISCUSSION
The different farms employed quite different regimes during the spring flush period. The main factors contributing to the farmer’s decision to turn out their herd are based on weather conditions and the growth of their pasture. Some unusual weather occurred in the spring/summer months of 2006. March, April and May were colder than normal while June and July were unusually hot, with temperatures up to 36.5 °C. May was a very wet month with more than double the average May rainfall. Bertoni et al. [2] also noted that climate change has a fluctuating effect on milk yield and composition, especially during hot and humid periods. It is possible that such effects had an influence in the current study, especially in the later samples.
While significant changes in composition and behaviour occurred during the course of this study, there were no wild swings in any of the factors measured, and certainly no evidence to suggest that any of the milk samples would have been unsuitable for processing. In no case did the milk fail to coagulate with acid or chymosin, neither did any samples form exceptionally weak gels, and there were no exceptionally long RCT results. Hence, the study fails to support the “spring flush phenomenon” with respect to coagulated dairy products. However, there were only a limited number of samples collected during this study and it is feasible that the phenomenon may have been missed. It should also be noted that different regimes were used by the farmers in the light of modern husbandry practices, which may have removed or moderated any of the more dramatic changes which might have occurred in earlier years. In the past, there would have been more uniformity of farming methods with the whole herd moving out to pasture in the spring. In this study, each farm had its own schedule for allowing the cows outside, based on the individual yield of different cows, the pasture characteristics, amount of pasture, use of feed supplements and farmers’ individual preferences. A key strength of this study is that the milk samples studied were true commercial milk for processing. However, the disadvantage was that the authors were unable to exercise any control over herd feeding and management during the experiment.
According to the statistical analysis, there is little evidence of changes in the data due to the stage of spring flush per se. The date of collection, however, did have a significant effect on the data, suggesting that seasonal factors other than specifically the turning out to spring pasture are important. It is well known that the season has an effect on the quality and composition of milk through dietary, stage of lactation and other effects. Seasonal variation clearly influences cheesemaking properties. For example, in the Parmigiano-Reggiano zone in Italy, two typical types of cheeses are produced according to season. Parmigiano-Reggiano is produced in spring and summer, while Vernengo is produced in winter [1]. Grandison et al. [7] found that the composition and coagulating properties of renneted Friesian milk were significantly altered during the change to spring grazing. The RCT was found to be correlated to fat, calcium concentration and pH, among other attributes. In the current study fat correlates negatively with RCT, whereas Grandison et al. [7] found the correlation to be positive, suggesting that the relationship is not causal. Green and Grandison [8] noted that dietary factors can have a marked effect on the renneting behaviour of milk and suggest that curd firmness is greater during pasture feeding than when the animals are housed indoors. The curd firmness of the rennet gels was not measured in this study, but the strength and viscosity of acid gels were increased after turning out. Grandison et al. [7] also found many other correlations between the chemical characteristics of milk. They indicate that the casein concentration increased throughout the study, lactose also increased but fat initially decreased and then increased. A similar trend was observed for fat and protein in this study, but the lactose decreased throughout spring flush.
In summary, the current study demonstrates some changes in milk composition and coagulation properties during the spring period, but no particular evidence of processing problems related to the spring flush.
Acknowledgments
The authors would like to thank the Barham Benevolent Foundation for its financial support and P. Chatfield for advice on statistical analysis. They are grateful to J. Hanks (VEERU) for organising milk collections and to the various farmers for providing milk samples.
References
- Addeo F., Neviani E., Mucchetti E., Ledda A., From milk to the cheese: technological criteria determining the quality of cheese, in: Flamant J.C., Gabina D., Espejo Diaz M. (Eds.), Basis of the Quality of Typical Mediterranean Animal Products, European Association of Animal Production No. 90, Wageningen Pers., Wageningen, The Netherlands, 1998, pp. 105–114.
- Bertoni G., Calamari L., Maianti M.G., Producing specific milks for speciality cheeses, Proc. Nutr. Soc. 60 (2001) 231–246 [PubMed].
- Boland M., Influences on raw milk quality, in: Smit G. (Ed.), Dairy Processing: Improving Quality, Woodhead Publishing, Cambridge, UK, 2003.
- Dairy Facts and Figures 2004, Milk development council, Cirencester, UK, 2005.
- Davies D.T., White J.C.D., The determination of calcium and magnesium in milk, J. Dairy Res. 29 (1962) 285–296.
- Ford G.D., Grandison A.S., Effect of size of casein micelles on coagulation properties of skim milk, J. Dairy Res. 53 (1986) 129–133 [CrossRef].
- Grandison A.S., Ford G.D., Owen A.J., Millard D., Chemical composition and coagulating properties of renneted Friesian milk during the transition from winter rations to spring grazing, J. Dairy Res. 51 (1984) 69–78 [CrossRef].
- Green M.L., Grandison A.S., Secondary (non-enzymatic) phase of rennet coagulation and post-coagulation phenomena, in: Fox P.F. (Ed.), Cheese: Chemistry, Physics and Microbiology, Chapman and Hall, London, UK, 1993, pp. 101–140.
- Lin M.-J., Lewis M.J., Grandison A.S., Measurement of ionic calcium in milk, Int. J. Dairy Technol. 59 (2006) 192–199 [CrossRef].
- MDC Dairy Statistics: an insider's guide, Milk development council, Cirencester, UK, 2007.
- Storry J.E., Grandison A.S., Millard D., Owen A.J., Ford G.D., Chemical composition and coagulating properties of renneted milks from different breeds and species of ruminant, J. Dairy Res. 50 (1983) 215–229 [CrossRef].
- Tsioulpas A., Lewis M.J., Grandison A.S., Effect of minerals on casein micelle stability of cows' milk, J. Dairy Res. 74 (2007) 167–173 [CrossRef] [PubMed].
- Walstra P., Jenness R., Dairy Chemistry and Physics, Wiley & Sons, New York, USA, 1984
All Tables
Repeated measures analysis to test the effect of date and stage on variation of measured parameters.
All Figures
![]() |
Figure 1. The TDC and ionic calcium content of milk from five farms throughout spring flush. |
In the text |
![]() |
Figure 2. The RCT (measured instrumentally) of milk from five farms throughout spring flush (mean of two measurements) and stirred gel viscosity (complex modulus) of gels made from milk from five farms throughout spring flush. |
In the text |
![]() |
Figure 3. Relationship between ionic calcium and pH during spring flush. |
In the text |